光技術情報誌「ライトエッジ」No.13(1998年7月発行)
Advanced Solid-State Laser Optical Society Of America
(1998年2月)
Development of a hiigh-power,high-repetition rate, diode-phmped, deep UV Iaser system
A Finch, Y. Ohsako, J. Sakuma
USHIO Institute of Technology (Boston Research Laboratory)
135 South Road, Bedford MA 01730
Tel: 781 687 9127, e-mail: afinch@ushio.com
K. Deki, M. Horiguchi
USHIO Institute of Technology (Tsukuba R &D Laboratory)
5-2-4 Tokodai, Tsukuba, lbaraki 300-26 Japan
Y. Mori, T Sasaki
Dept of Electrical Engineering, Osaka University
2-1 Yamadaoka, Suita, Osaka 565, Japan
K. Wall, J. Harrison*, P.F. Moulton
SEO Boston,
135 South Road, Bedford MA 01730
J. Manni
JGM Associates, Inc.
6 New England Executive Park, Suite 400
Burlington, MA 01803
* Now at: OptoPower Corp, 3321 E. Global Loop, Tucson, Az 85706
Introduction
There has been great interest in the development of UV and deep-UV Iaser systems fot industrial, scientific, and medical applications. The achievement of high-average power, deep-UV radiation via the fourth and fifth harmonic generation of diode-pumped 1-µm lasers is of particular importance for the semiconductor photolithography industry, since this provides part of the pathway to 193-nm generation. One of the difficulties in the development of high power UV or deep-UV is the availability of suitable nonlinear crystals and their thermal management due to linear and/or nonlinear absorption. Recently CLBO has proven to be a promising candidate in this area. Development work with this material has been reported by Yap et al.[l], Stamm et al.[2], and recently 192-nm generation using CLBO has been demonstrated by Deki et al.[3].
In many industrial applications, high repetition rate, as well as high average power, is an important issue in terms of throughput or total performance. The majority of the high power UV generation has been confined to 100-Hz repetition rates. UV generation. using diodepumped Ndbased systems, has been reported at higher repetition rates by Masuda et al.[4] but only with single Watt-level power generation in the UV. In this paper, we report on a high-power, high-repetition, all-solid-state, UV laser system generating 6.6W at 262nm and 2.0W at 209nm using CLBO. The system utilizes a diode-pumped Nd:YLF 1047-nm MOPA system which produces 37W CW and 29W Q-switched TEM 00 output power at a 5-kHz repetition rate.
Nd:YLF MOPA system
The system under development is shown in Figure 1. A basic building block of the MOPA is a multipass gain module developed and manufactured by SEO Boston[5]. Each gain module comprises a 2.8-cm Nd:YLF crystal slab that is side-pumped by two fiber-lensed, 20-W diode laser bars. The bars are offset on opposing sides of the Nd:YLF crystal, generating a gain sheet inside the slab. The gain is accessed by the multipassing the 1047-nm beam (five passes in total) using external mirrors located close to either end of the AR-coated slab. Additional spherical and cylindrical mirrors in the resonator create an elliptical beam shape inside the Nd:YLF slab.
The pump geometry of the gain module provides high distributed gain, coupled with low pumpinduced lensing (of order lm in both x and y directions) and low pump-induced birefringence (relative to the strong intrinsic YLF birefringence). Such properties are ideal for high-power oscillators, and resonators incorporating a single gain module have generated in excess of 14-W TEM∞ 1047-nm CW power. For similar reasons the design is also ideal for use as a high gain amplifier. Small signal gains of over 4 have been measured for single pass through one gain module. Operated as a CW power amplifier 50% extraction efficiency of the available power has been demonstrated.
The system used in these experiments incorporates two gain modules amplifying a standing wave acousto-optically, Q-switched oscillator (a slightly modified SEO MPS-1047 resonator design). The CW output curve for this MOPA system is shown in Figure 2 as function of input power to the second amplifier stage and diode current applied. As can be seen the MOPA is capable of producing 37W CW. When Q-switched at a repetition rate of 5kHz, the system generates 29-W average power. The entire MOPA system occupies an 18-inch by 24- inch breadboard. Included on Figure 1 are the output powers of each stage of the MOPA system.
Particular care was taken to mode match the vertical beam dimension of the input beam into each gain module gain sheet and also to accommodate any lensing present in amplification stage. This allowed TEM∞ output of exceptional beam quality to be produced. A measurement of the output beam with a Spiricon M2 -101 beam measurement system yielded M2 values of 1.07 and 1.10 in the horizontal and vertical directions. Based on the accuracy of this instrunent we can assume that M2 values of the amplified beams are well less than 1.2. Higher powers can be obtained from the MOPA system by the simple addition of more gain modules and beam relay image lenses.
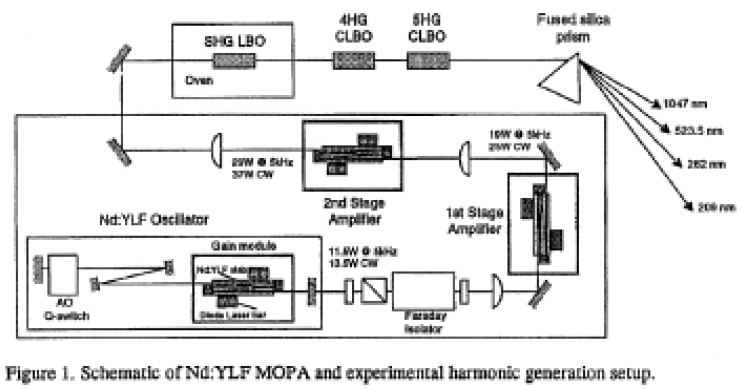
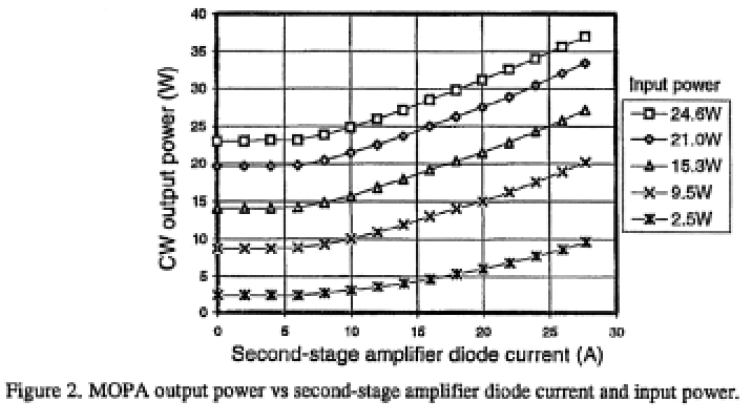
Harmonic generation
Due to an additional uncoated beam shaping optic, the available power delivered to the harmonic chain from the MOPA at 5-kHz Q-switched operation was 26W with a pulse width of 20 ns (FWHM). The beam was collimated only in the vertical (y) direction with a positive cylindrical lens. A temperature tuned, non-critically phase-matched LBO crystal was used to frequency double the Nd:YLF MOPA output to the green (523.5nm). The spot size of the 1047-nm beam at the LBO crystal was wx=150µm by wy=250µm. The generated average power at 523.5 nm was 14. 1W useful output, with an additional 0.4W backreflected power caused by poor AR-coating. The M2 value was measured to be less than 1.2.
The green output was frequency doubled in CLBO to generate fourth harmonic at 262 nm without additional focusing optics. The CLBO crystals were grown by USHIO using a top seeded growth method. We used two uncoated crystals cut for type- 1 phase matching with lengths of 15 and 20 mm. The measured average power at 262 nm was 4.6W and 5.1W for the 15-mm and 20-mm length crystals respectively. Taking into account reflection losses at the surfaces of the harmonicseparating prism and at the fourth harmonic (4HG) crystal surface, this corresponds to 6.1W and 6.6W generated internally inside the crystal respectively. Power readings were taken only after they had stabilized i.e. after the crystal had thermally equilibrated (which typically was after a few minutes). Figure 3 shows the average power and energy conversion efficiency for the fourth harmonic generation as a function of input power at 523.5nm. The conversion efficiency of input fundamental to fourth harmonic output was 25%.
The 262-nm light was then combined with residual 1047-nm light in a 10-mm long CLBO cut for type-1 phase matching. The generated fifth harmonic (5HG) output at 209 nm was 2.0W with the 15-mm long 4HG crystal and 1.5W with the 20-mm crystal. We suspect that the Iower fifth harmonic power obtained with the 20-mm 4HG crystal was due to larger walk off in this crystal. This causes a reduced spatial overlap of the 1047-nm and 262-nm light in the 5HG crystal. The overall optical conversion efficiency from fundamental to fifth harmonic was 7.6%.
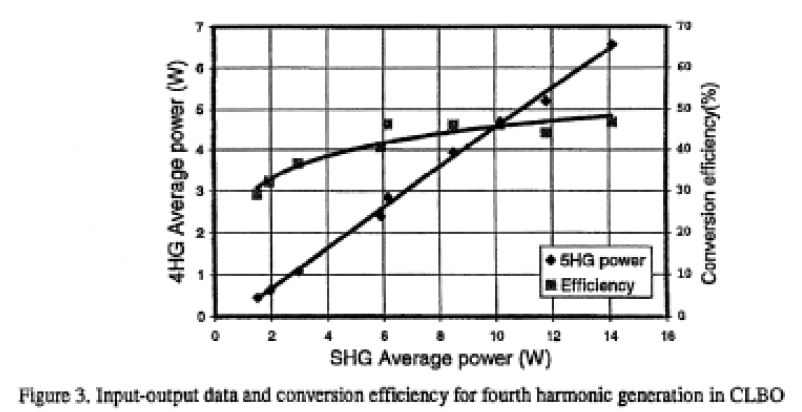
Damage was observed in the CLBO near the output surface of the 20-mm length 4HG crystal and also the 5HG crystal, only when the UV power at 262-nm exceeded 5W. This fact indicates that the damage was caused by the 262-nm light generated in the CLBO. Its damage threshold at 5kHz operation is estimated to be 3.4 J/cm2 .
Conclusion.
In summary, we have demonstrated a high-repetition-rate, multi-watt deep UV laser source based on a high power Nd:YLF MOPA system using LBO and CLBO. We have obtained 6.6W average power at 262 nm and 2.0W at 209 nm. These powers represent, to our knowledge, the highest reported UV powers so far in the kHz-pulse repetition rate regime. Future improvements to this system will include single longitudinal mode operation, and the frequency conversion to 193nm.