光技術情報誌「ライトエッジ」No.42(2015年05月発行)
Biosensors and Bioelectronics
(2015.3)
Highly selective and sensitive detection
of β-agonists using a surface plasmon
resonance sensor based on an alkanethiol
monolayer functionalized on a Au surface
Suhermana, b, Kinichi Moritac, Toshikazu Kawaguchia
aDivision of Environmental Materials Science, Graduate School of Environmental Science and Section of Materials Science, Faculty of Environmental Earth Science, Hokkaido University, Sapporo 060‐0810, Japan
bDepartment of Chemistry, Faculty of Mathematics and Natural Sciences, Universitas Gadjah Mada, Sekip Utara Kotak Pos 21 BLS, Yogyakarta 55281, Indonesia
cNew Business Development Office, USHIO INC., 6-1 Ohtemachi 2-chome, Chiyoda-ku, >Tokyo 100-8150, Japan
ARTICLE INFO: Article history: Received 3 June 2014
Received in revised form 21 August 2014
Accepted 22 August 2014
KEYWORDS: Surface plasmon resonance
β-agonist compounds
Indirect competitive inhibition
immunoassay
ABSTRACT
Immunosensor surfaces for surface plasmon resonance (SPR) have been constructed using a functionalized succinimidyl propanethiol monolayer as a linker to immobilize β-agonist protein conjugates on a Au surface. Because β-agonist is a small molecule, an indirect competitive inhibition immunoassay was used for detection. The lowest detection limits for ractopamine and salbutamol were 10 ppt (10 pg mL-1) and 5ppt (5pg mL-1), respectively. The fabricated immunosensor surface can be used again for detection after regeneration in 0.1 M sodium hydroxide. It was found that the same sensor surface could be reused for performing over 100 rapid immunoreactions. Moreover, one immunosensingregeneration cycle requires only 600 s. The fabricated immunosensor surfaces were characterized using SPR and scanning tunneling microscopy observation. In the kinetic study of the indirect competitive immunosensing inhibition, the affinity constant (K1) of salbutamol antibody was smaller than the K1 of ractopamine antibody. Compared to a previous study of clenbuterol detection, it was concluded that the high K1 was coupled with low sensitivity. In the selectivity study, both immunosensor surfaces provided >90% of confidence level for the specific detection of β-agonist compounds. The fabrication of highly selective and sensitive sensor surfaces for detecting β-agonist compounds was confirmed.
© 2014 Published by Elsevier B.V.
1. Introduction
β-agonists are usually used as drugs for the treatment of respiratory diseases and asthma (Crescenzi et al., 2001). However, due to their potential roles in reducing animal fat levels and increasing the amount of muscling in livestock, β-agonists were used in animals as growth promoters to increase the daily weight gain (Shishani et al., 2003). β-agonists compounds can be easily stored in human tissues after meat consumption, and result in many serious health problems with symptoms such as palpitations, tremors and tachipnoea (Brambilla et al., 2000). Furthermore, more than 150 countries have strictly banned the use of βagonists in stockbreeding, including China, Japan and Europe, due to their negative side effects in the human body (Liu et al., 2012). Inspection services for food safety are particularly interested in the three main β-agonists, i.e., clenbuterol, ractopamine and salbutamol. Thus, ractopamine and salbutamol are the targets of this study. However, they have similar chemical structures (i.e., they are phenyl ethanolamine compounds) (Lin et al., 2013) and almost the same molecular weight (230–300 Da). In the past studies, analytical methods using high-performance liquid chromatography (Zhang et al., 2004), liquid chromatography/tandem mass spectrometry (Antignac et al., 2002; Li et al., 2010; Juan et al., 2010; Wu et al., 2014) and gas chromatography–mass spectrometry (He et al., 2007) have been reported for determining βagonists residues. Although these chromatographic approaches can provide satisfactory reproducibility, they are time consuming, require high sensitivity and less appropriate for onsite usage due to elaborate sample preparation (Lin et al., 2013).
Biosensors effectively detect chemical and biological analytes. Optical biosensors based on surface plasmon resonance (SPR) provide high sensitivity without using molecular labels. Thus, the sample preparation time can be minimized. To date, SPR biosensors have been reported in various applications such as medical diagnostics, drug discovery, environmental monitoring, food safety and homeland security (Homola, 2008; Cooper, 2002; Torun et al., 2012; Farré et al., 2007; Piliarik et al., 2009 ; Petz, 2009 ; Kawaguchi et al., 2008; Shankaran et al., 2007). SPR is a mass transducer and able to monitor the dielectric constant change as the resonance angle shift. In our SPR system, the limit of detection (LOD) for the resonance angle shift is 0.01 mdeg (0.1 RU). Thus, a mass change of 0.01 ng cm-2 due to the adsorption of a protein can be detected. Among the mass transducers, the SPR sensors show the highest sensitivity. To add selectivity to the highly sensitive SPR sensor, immunoassay is usually used.
To date, various methods of immunosensor surface fabrication have been reported. In our previous study, clenbuterol equipped with an amine group was directly immobilized onto a succinimidyl propanethiol monolayer on the sensor surface by amide coupling reaction. However, ractopamine and salbutamol do not have a functional group that can form a steady chemical bond to thiol compounds. Thus, we choose to immobilize the ractopamine– bovine serum albumin (RCT–BSA) conjugate and the salbutamol– horseradish peroxidase (SAL–HRP) conjugate onto a succinimidyl propanethiol monolayer. For the immobilization of the protein conjugate, the physical adsorption method has been proposed (Gobi et al., 2007; Tsai et al., 2011). This method exploits the hydrophobic interaction between proteins and the Au surface. However, this interaction is weak, and therefore, the surface could be regenerated up to 30 times by using glycosine–HCl solution (Gobi et al., 2007).
Herein, we propose an immunosensor surface comprising a self-assembled alkanethiol monolayer on a Au chip for highly sensitive and selective detection of ractopamine and salbutamol. A succinimidyl-terminated propanethiol monolayer is prepared from dithiobis(succinimidyl) propionate (DSP) by self-assembling. Subsequently, protein conjugates can be instantly immobilized by replacing the succinimidyl group with a NH2 group of a protein in a neutral buffer by a single-step reaction. We discuss protein-conjugate-immobilized sensor surfaces by using scanning tunneling microscopy (STM) observation. We also propose selectivity test procedures for multiple-target detection in indirect competitive inhibition immunosensing.
2. Material and methods
2.1. Materials
KOH, salbutamol sulfate, and ethanol were obtained from WAKO, Japan, while NaOH from Junsei Chemical Co., Ltd., Japan. DSP and phosphate buffer saline (PBS) were purchased from Sigma-Aldrich, USA. Ractopamine–BSA was purchased from Bioss, Inc., USA. Methanol was obtained from Dojindo, Japan. Ractopamine hydrochloride and monoclonal mouse IgG antibody (Ab) against clenbuterol (CLB) were purchased from LKT Laboratories, Inc., USA. Monoclonal mouse IgG Ab against ractopamine (RCT) was purchased from Acris Antibodies, USA. Salbutamol–HRP was purchased from Aviva System Biology, USA, and polyclonal sheep IgG Ab against salbutamol (SAL) was ordered from GenWay Biotech, Inc., USA. Refractive index matching fluid (refractive index=1.518) was obtained from Cargille Labs, USA. All chemicals were of reagent or higher grade, and Millipore water (18.2 MΩ cm) was used throughout the experiments.
2.2 Preparation of Au substrates
Glass substrates (BK7 type, 20 mm x 13 mm x 0.7 mm from Matsunami Glass Ind., Ltd., Japan) were sonicated in soap water (10% Contrad 70 detergent from Fisher). After rinsing with sufficient water, the glass substrates were dried with pure nitrogen gas. To make the surface hydrophilic, the glass substrates were cleaned using plasma at 15 W under 2.0–2.5 Pa. Subsequently, Au (50 nm) was sputtered onto the glass chips under 2.0–2.5 Pa. The prepared Au chips were loaded into the SPR sensing system immediately after excimer ashing pretreatment (172-nm incident light) for 2 min.
Reported surface densities of monolayers were corrected for the surface roughness of the Au chip (δ=1.50), which was calculated from the reduction charge density of Au–O in the cyclic voltammogram in 0.1 M sulfuric acid.
2.3. Scanning tunneling microscopy (STM)
STM experiments were conducted using a NanoScope STM III (Digital Instruments, USA) operating in a constant current mode (a few hundred pico-ampere) with a bias voltage typically between 0.5 and 1 V. For imaging, Pt/Ir (80/20) mechanically cut tips (diameter=0.25 mm, Bruker, USA) were used for surface scanning.
2.4. Surface plasmon resonance (SPR) sensing
SPR experiments were conducted on an SPR-670 instrument (Nippon Laser Electronics, Japan), equipped with a completely automated flow system comprising a plunger pump and an injector. The Au chip was mounted on a semicylindrical prism with a refractive-index-matching liquid. Red light (670 nm) emitted from a Ni–Cd laser was reflected at the Au-coated glass plate at attenuated total reflection angles, and the reflected light intensity was recorded using a charge-coupled device camera. The reflectance angle, at which the light intensity was minimum (SPR angle), was recorded with time. All the experiments were conducted in an air-conditioned room (i.e., 25 °C).
2.5. Sensor surface fabrication
Fig. 1 shows SPR sensorgram of the sensor surface fabrication. First, self-assembly of the alkanethiol monolayer was conducted by injecting a 5-mM DSP methanolic solution over an unmodified Au surface for 40 min at a 5 µL min-1 flow rate immediately after the resonance angle stabilized during the solution flow (Fig. 1(i)). With this self-assembly process, the Au surface became completely covered by a succinimidyl-terminated propanethiol monolayer. Second, the SPR running solution was changed from methanol to PBS (pH 7.4). This change caused an abrupt shift in the resonance angle because of the refractive index difference between the methanol and PBS solutions. After the resonance angle restabilized, protein conjugates (PBS solution containing 50 ppm of ractopamine–BSA or salbutamol–HRP conjugates) were injected for 40 min (Fig. 1(ii)). With this process, the succinimide group was replaced to provide β-agonist-protein conjugates.
Although protein conjugates evenly flowed over the succinimidyl-terminated propanethiol monolayer on the sensor chip, unreacted succinimide groups remained. To prevent the occurrence of nonspecific adsorption, ethanolamine (PBS solution containing 1mg mL-1 blocking agent) was injected for 40 min to protect the remaining succinimide groups (Fig. 1(iii)). Unreacted succinimide groups were completely blocked by ethanolamine. Then, the sensor surface was used for detecting β-agonist compounds.
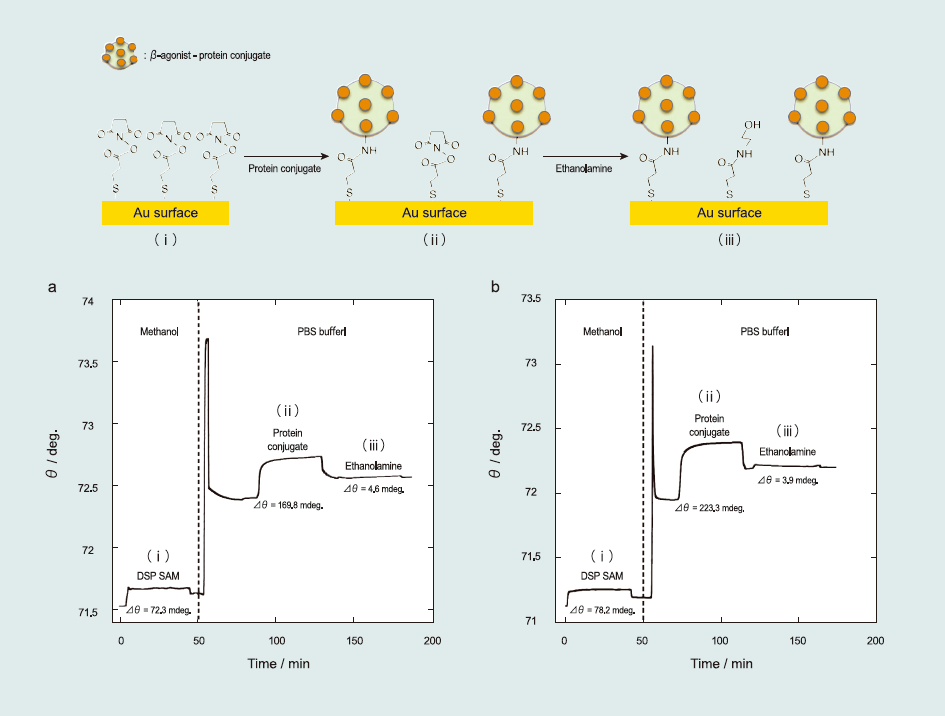
Fig.1 SPR sensorgram of the fabrication of the sensor surface: (i) self-assembly of the succinimidyl-terminated propanethiol monolayer, (ii) immobilization of the βagonistprotein conjugates (ractopamine–BSA and salbutamol–HRP), and (iii) blocking of the unreacted succinimidyl-terminated propanethiol with ethanolamine.
2.6. Immunoassays
The immunoassay format of indirect competitive inhibition was employed for detecting β-agonist compounds, as previously described (Soh et al., 2003; Kumbhat et al., 2006; Miura et al., 2007). As a mass transducer, SPR observes the dielectric constant changes due to the adsorption of heavyweight antibodies rather than lightweight antigens (β-agonists) at the interface. Thus, larger signal responses are expected in this immunoassay.
In this study, the Ab solution (PBS solution containing 1 µ gmL-1 of Ab) was first premixed with a sample solution (PBS solution containing β-agonist compounds) before injection into the sensing system. As the mixture flows over the sensor surface, the SPR senses the dielectric constant changes due to the binding of unreacted Ab to the antigen immobilized on the sensor surface. After detection, Ab was removed from the sensor surface by using 0.1 M NaOH. Hence, the sensor surface was reused for detection. Moreover, we confirmed reproducibility using different sensor chips (N>3), and each plot was averaged within 10% experimental error bars.
3. Results and discussion
3.1. Detection of β-agonists
Fig. 2 shows the representative sensorgrams of the immunoreaction with anti-ractopamine (a) and anti-salbutamol antibodies (b) to the sensor surface.
First, since the sample solution was injected into the SPR system, the resonance angle gradually increased due to Ab adsorption onto the sensor surface and stabilized after 200 s. The resonance angle difference between 100 and 300 s, determined as the total angle shift (Δθ) of the immunoreaction, was used to calculate the amount of adsorbed species. From SPR experiments, Δθ0 for 1 µg mL-1 RCT and 1 µg mL-1 SAL were determined as 32.33±1.62 and 5.73±0.10 mdeg, respectively. The lower value obtained for SAL Ab indicated that it has low affinity to the sensor surface. Second, after the immunoreaction, the sensor surface was regenerated using 0.1 M NaOH. After the regeneration process, the resonance angle returned to its initial value, indicating that Ab was completely removed from the sensor surface. The sensor surface could be used for detection more than 100 times without any damage, although the repetition time of the protein-conjugate physically immobilized sensor surface was limited to 30 times. One immunosensing-regeneration cycle requires only 600 s. Thus, the immunoreaction was repeatedly examined using the same sensor surface for multiple sensing.
The target β-agonists were detected by indirect competitive inhibition immunoassay. Fig. 3 shows the β-agonists solution concentration dependence. From the sigmoid-type curve, the calculated LOD was 5–10 ppt (pg mL-1)—the highest sensitivity reported among β-agonists detection studies. Moreover, this result is comparable to a previous study on the clenbuterol detection using an alkanethiol monolayer functionalized on a Au surface, which yielded LOD values of 3–7 ppt (Suherman et al., 2014).
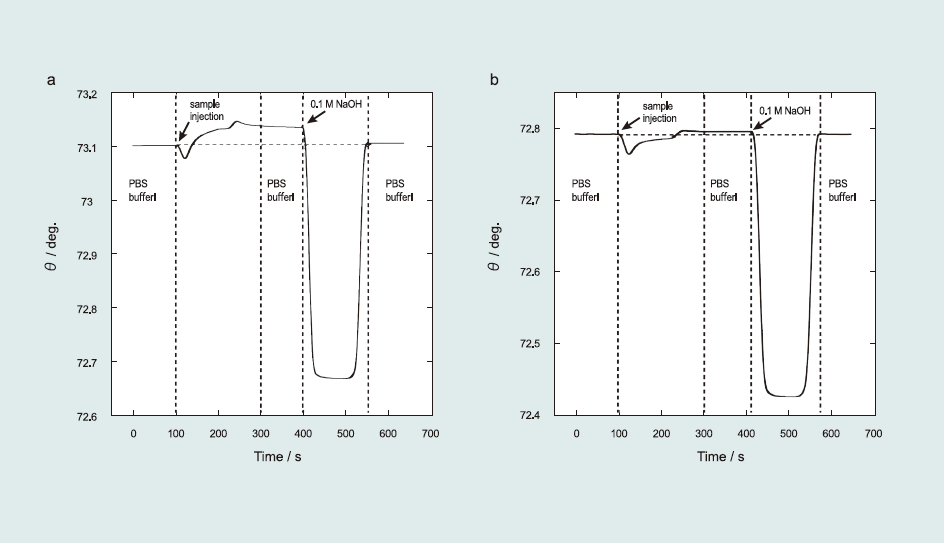
Fig.2 SPR sensorgrams of the detection of (a) ractopamine and (b) salbutamol. The flow rate was 100 µLmin-1, PBS (10% ethanol) solution was used as the running solution, and 0.1 M NaOH aqueous solution was used to regenerate the sensor surface.
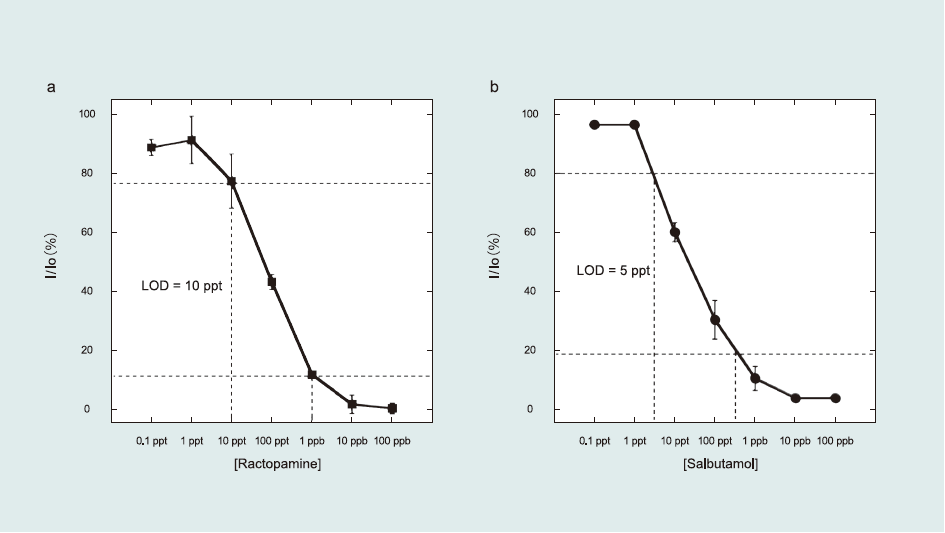
Fig.3 Sigmoid curves of β-agonists solution concentration dependence obtained using the indirect competitive inhibition immunoassay. The inhibition rate was calculated from the angle shift in the presence (I)/absence (I0) of β-agonists. In the premixing process, β-agonists Ab was mixed with a series of antigen concentrations. The incubation time was 2 min.
3.2. Quantitative analysis
Following previously reported kinetic analyses (Kumbhat et al., 2006; Miura et al., 2007; Sakai et al., 1999, 1998), the affinity constant (K1) of Ab to the β-agonist (antigen)-immobilized sensor surface and the maximum angle shift of the SPR after Ab injection (Δθ0,max) were determined using a Langmuir-type adsorption model (Fig. 4(a) and (b)). The detailed kinetic equation is described in the Supporting information (Eqs. S1–S8).
K1 and Δθ0,max were calculated as 1.69-107 M-1 and 120.2 mdeg for RCT and 0.79 x 107 M-1 and 11.4 mdeg for SAL, respectively. From comparison of K1 for RCT and SAL, the lower K1 of SAL reveals its higher sensitivity (5 ppt) than that of RCT (10 ppt). Therefore, it can thus be concluded that lower K1 corresponds to higher sensitivity.
K2 values with constant α for RCT and SAL were also calculated as 1.20 x 1010 M-1 and 2.93 x 109 M-1, respectively (Fig. 4(c) and (d)). Here, K2 values were higher than K1 for both RCT and SAL. Antibodies preferentially bind free β-agonists in solution than βagonists immobilized on sensor surfaces, which results in lower K1. In addition, although the kinetic analyses provided K1 < K2, sensitivity of the sensor surface was high(i.e., 5–10 ppt) in detecting β-agonist compounds. In summary, higher sensitivity in detecting β-ago nists is related to lower K1 of Ab to antigen immobilized on the sensor surface.
Fig. 5 shows STM images of sensor surfaces comprising a protein -conjugates-terminated propanethiol monolayer. For the RCT-BSA conjugate, one β-agonists-protein conjugate complex occupied approximately 100 nm2. The surface concentration was directly determined by the number of conjugates in the image, calculated to be 1.7 x 10-12 mol cm2. Moreover, considering the value of resonance angle shift (Δθ) after protein conjugate injection into the SPR system, (169.8 mdeg) (Fig. 1(a)), the surface concentration of the RCT–BSA conjugate was 2.6 x 10-12 mol cm-2. Similarly, considering that one molecule occupied approximately 36 nm2, the surface concentrations of the SAL– HRP conjugate were 4.6 x 10-12 mol cm-2 (STM) and 5.6 x 10-12 mol cm-2 (SPR). Surface concentrations calculated using SPR and STM images coincided well.
From β-agonists Ab injections (Fig. 2(a) and (b)), we could determine the amount of reacted Ab compared to the surface concentration of β-agonists protein conjugates immobilized on the surface. Δθ of 1 µg mL-1 Ab injections were 32.33±1.62 mdeg (RCT) and 5.73±0.10 mdeg (SAL), which corresponds to 9.72 molecules/2500 nm2 (RCT) and 1.23 molecules/2500 nm2 (SAL) whereas the surface concentration of β-agonists-protein-conjugates calculated from the STM images (Fig. 5(b) and (c)) were approximately 10 molecules/2500 nm2 (RCT) and 18 molecules/ 2500 nm2 (SAL). Therefore, the Ab to β-agonists-protein-conjugates ratios were 1.0 for RCT and 0.07 for SAL. Thus, the low ratio of SAL directly correlates with low K1.
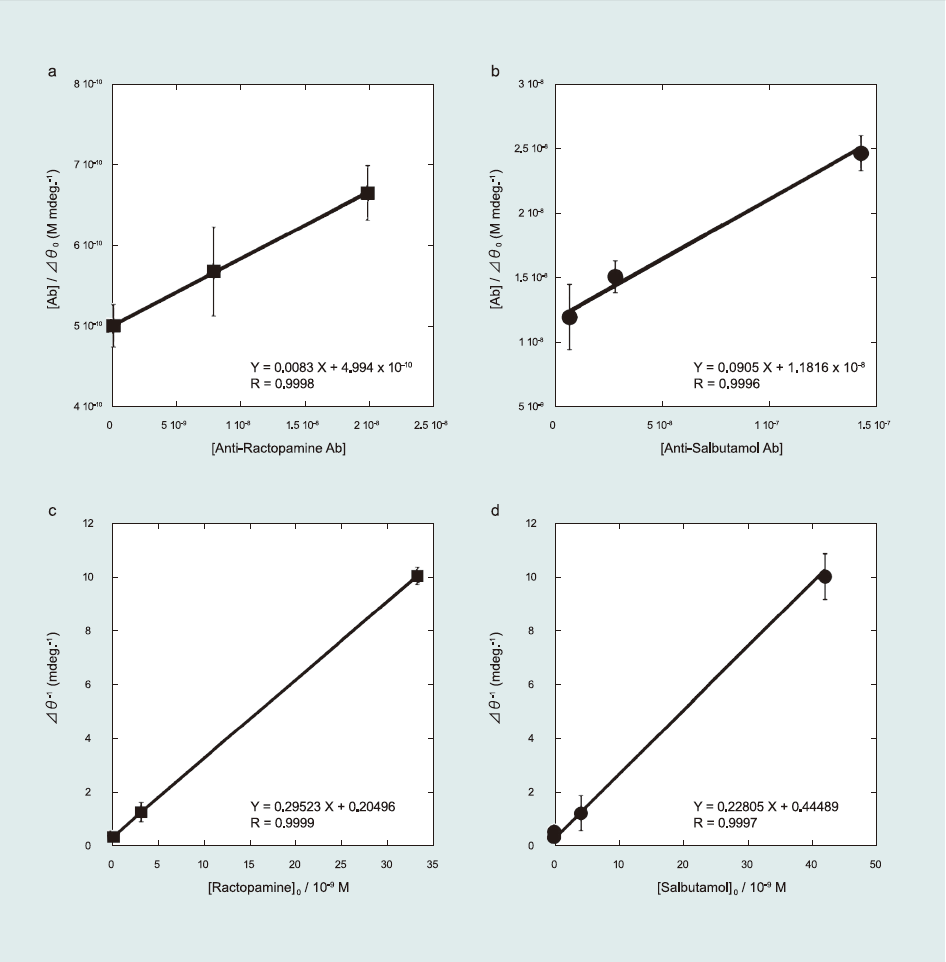
Fig.4 Kinetic study of the β-agonists detection. Ab concentration dependence for (a) ractopamine and (b) salbutamol. Antigen concentration dependence for (c) ractopamine and (d) salbutamol.
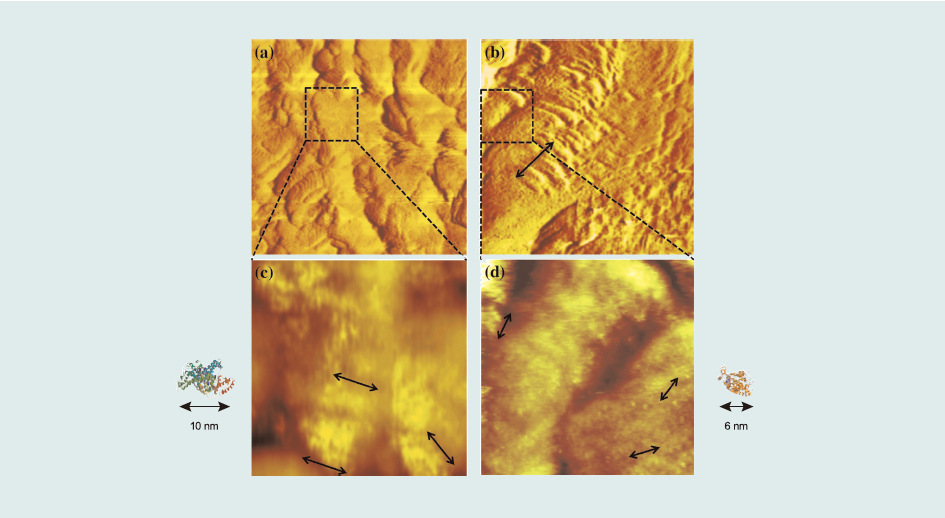
Fig.5 STM images of a 200nm x 200nm area of the sensor surface: (a) ractopamine–BSA conjugate sensor surface, and (b) salbutamol–HRP conjugate sensor surface. STM images of a 50nm x 50 nm area of the sensor surface: (c) ractopamine–BSA conjugate sensor surface, and (d) salbutamol–HRP conjugate sensor surface.
3.3. Selectivity study
To study selectivity of the sensor surface in multiple-target detection, we used cross-reactivity and multiple Ab injection experiments(Ab matrices test). For the cross-reactivity study, we compared the total resonance angle shifts of single antibody injection and mixed antibodies injection (target Ab in the presence of interfering Abs).
Fig. 6(a)–(d) exhibit the effect of Ab matrices on the target analyte. For the RCT-based sensor surface, cross-reactivity provided a 91% confidence level as estimated from Δθ of 32.33±1.62 mdeg and 29.43±1.68 mdeg for single and mixed Ab injection (RCT Ab in the presence of CLB Ab and SAL Ab), respectively. For the SAL-based sensor surface, the selectivity study yielded 97% confidence level, which was calculated from Δθ of 5.73±0.10 mdeg and 5.56±0.10 mdeg for single and mixed Ab injection, respectively, indicating high selectivity of sensor surface against similar β-agonists Ab. We also confirmed the selectivity study by injecting multiple Abs on the sensor surface (i.e., RCT, SAL, and CLB Ab injection to the RCT and SAL sensor surfaces (Fig. S1 in Supporting information). We determined that the RCT and SAL sensor surfaces clearly distinguished between related Ab and others with approximately 90% confidence level.
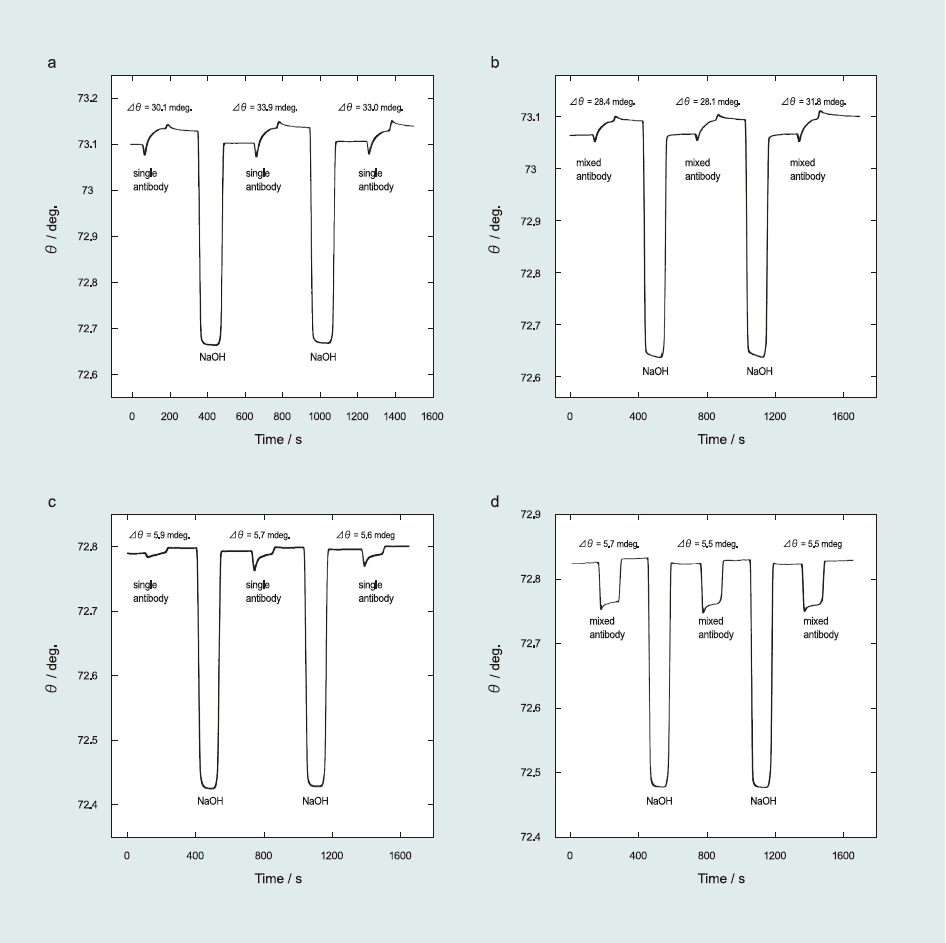
Fig.6 Selectivity study of β-agonist antibodies: RCT sensor surface against RCT Ab in the (a) absence and (b) presence of SAL Ab and CLB Ab; SAL sensor surface against SAL Ab in the (c) absence and (d) presence of RCT Ab and CLB Ab.
4. Conclusions
In this study, β-agonist-protein-conjugates covalently immobilized onto sensor surfaces were fabricated for detecting ractopamine and salbutamol. Therefore, a succinimidyl-terminated propanethiol monolayer was used as a linker molecule. The indirect competitive inhibition immunoassay was used for β-agonists detection. It was found that the lowest LOD was 5–10 ppt, which indicated remarkably high sensitivity compared to previous studies. Furthermore, this immunosurface could be regenerated using 0.1 M NaOH. One immunosensing-regeneration cycle required only 600 s, whereas the same surface could be used for >100 immunoreactions although the sensor surface fabricated using physical adsorption could be reused up to 30 times.
From the SPR and STM studies, the surface concentration of RCT–BSA and SAL–HRP conjugates were approximately 2 x 10-12 mol cm-2 and 5 x 10-12 mol cm-2, respectively. It was also determined that one RCT–BSA conjugate reacted to one RCT Ab in 1 µg mL-1 RCT Ab solution, whereas one SAL–HRP conjugate was associated with 0.07 SAL Ab. Consequently, SAL Ab showed a low affinity constant, K1. From the kinetic analysis, a low K1 revealed a high sensitivity of the detection process.
For multiple targets detection, we conducted a test using Ab analog matrices, which determined that the fabricated systems have a good selectivity at >90% confidence level for specific Ab. Thus, we concluded that the fabricated immunosensor surfaces are highly selective and sensitive.
Acknowledgments
The authors acknowledge the Hitachi Scholarship Foundation for their support and assistance.
Appendix A. Supplementary information
Supplementary data associated with this article can be found in the online version at http://www.sciencedirect.com/science/article/pii/S0956566314006514